Analytical Frameworks: Differential Equations in Aerospace Engineering




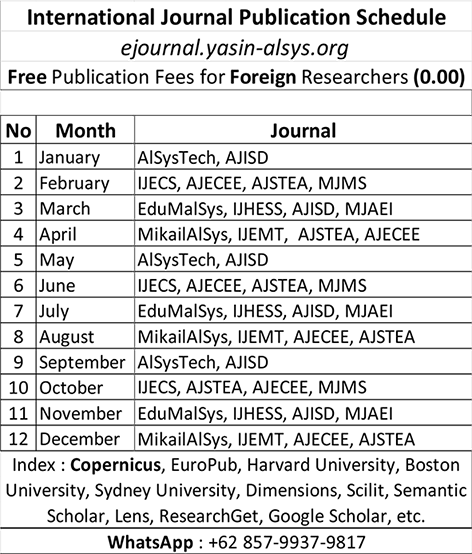
Please do not hesitate to contact us if you would like to obtain more information about the submission process or if you have further questions.
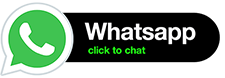
Abstract
This report explores the fundamental use of differential equations in understanding and modeling dynamic systems, tracing its roots for the contributions of mathematicians. Differential equations act as a basic platform for scientific and engineering research, providing insights into the dynamics of physical, and social systems. Their adaptability and associative applicability, especially in fields like environmental science and technology learning, highlight their main importance. The report dwells with specific applications in engineering, emphasizing their role in dynamic systems, control theory, and optimization. The definitions and types of differential equations are explained, showcasing their diverse characteristics. The historical evolution of differential equations, spanning centuries, underscores their continual refinement and application in various scientific disciplines. Moreover, the report presents hypothetical case studies illustrating the application of differential equations in the calculation of mass of fuel tank of rocket, time required by rocket to become triple its initial velocity. These examples showcase the practical utility of differential equations in enhancing precision and efficiency in space exploration. The advantages of application of differential equations in three-dimensional space are highlighted, emphasizing their role in realistic modeling, multidimensional dynamics, and scientific exploration. However, the report also contains certain drawback, such as increased complexity, computational intensity, and visualization challenges associated with three-dimensional systems. In conclusion, the study of differential equations remains vital for unraveling the complexities of the natural world and technological advancements, demonstrating their enduring significance in advancing human knowledge, healthcare, and innovation.
Downloads
Authors retain copyright and grant the journal right of first publication with the work simultaneously licensed under a Creative Commons Attribution-NonCommercial-ShareAlike 4.0 International License that allows others to share the work with an acknowledgement of the work's authorship and initial publication in this journal.
References
Anderson, J. D. Jr. (2005). Fundamentals of Aerodynamics. McGraw-Hill Education.
Battin, R. H. (1999). An Introduction to the Mathematics and Methods of Astrodynamics. American Institute of Aeronautics and Astronautics.
Bisplinghoff, R. L., Ashley, H., & Halfman, R. L. (1996). Aeroelasticity. Dover Publications.
Bong, T., & Kim, K. (2003). "Liquid Rocket Propulsion: Mathematical and Computational Modeling of Rocket Engine." Journal of Propulsion and Power, 19(1), 77-85.
Cohen, I. M. (2006). Fluid Mechanics. Academic Press.
Garris, C. A. (1999). Dynamic Systems: Modeling, Simulation, and Control. CRC Press.
Gustavsson, J. P. A., Holmborn, H., & Rosén, A. (2013). "Heat transfer analysis in a LOX tank during pre-cooling." Acta Astronautica, 90(1), 26-35.
Greenwood, D. T. (2010). Principles of Dynamics. Prentice Hall.
Humble, R. W., Henry, G. N., & Larson, W. J. (2011). Space Propulsion Analysis and Design. McGraw-Hill Education.
Huzel, D. K., & Huang, D. H. (1971). Design of Liquid Propellant Rocket Engines. American Institute of Aeronautics and Astronautics.
Kundu, P. K., Cohen, I. M., & Dowling, D. R. (2015). Fluid Mechanics. Academic Press.
Liu, H., Yu, Z., & Shen, H. (2016). "Numerical study of sloshing dynamics in a model of a liquid hydrogen tank for a space launcher." Aerospace Science and Technology, 51, 77-85.
Mattingly, J. D., Boyer, K. M., & von Ohain, H. (2014). Elements of Propulsion: Gas Turbines and Rockets. American Institute of Aeronautics and Astronautics.
McCormick, B. W. (1995). Aerodynamics, Aeronautics, and Flight Mechanics. John Wiley & Sons.
Park, J. H., & Choi, H. L. (2017). "An experimental study of liquid hydrogen sloshing in a cylindrical tank under microgravity." Acta Astronautica, 134, 288-297.
Rao, S. (2007). Engineering Optimization: Theory and Practice. John Wiley & Sons.
Rebrov, V. V., & Boiko, A. V. (2013). "Fluid dynamics in cryogenic tanks of space rocket systems." Acta Astronautica, 90(1), 148-153.
Sutton, G. P., & Biblarz, O. (2001). Rocket Propulsion Elements. John Wiley & Sons.
Stewart, W. L. (1966). "A Differential Equation for Free Turbulent Shear Flows." Journal of Fluid Mechanics, 24(2), 241-258.
Tewari, A. (2011). Modern Control Design with MATLAB and SIMULINK. John Wiley & Sons.
Wang, G., Pan, C., & Wang, X. (2020). "Thermal response of a liquid hydrogen tank subjected to cryogenic fluids injection." Cryogenics, 110, 103101.
Wilcox, D. C. (1993). Turbulence Modeling for CFD. DCW Industries.
Yechout, T. R., Morris, S. L., Bossert, D. J., & Shevell, R. S. (2003). Introduction to Aircraft Flight Mechanics: Performance, Static Stability, Dynamic Stability, and Classical Feedback Control. American Institute of Aeronautics and Astronautics.